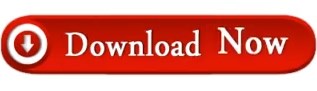


This amplification of the stimulus constitutes positive feedback that enhances the sensitivity of hearing by countering the loss of energy through the viscous dissipation that accompanies the motion of hair bundles and other structures through the liquids of the inner ear ( Gold, 1948). At the same time, however, the hair cells perform work by increasing the magnitude of their mechanical input. When sound reaches the cochlea, it elicits mechanical vibrations that are distributed to hair cells and transduced into an electrical response by their mechanoreceptive hair bundles.
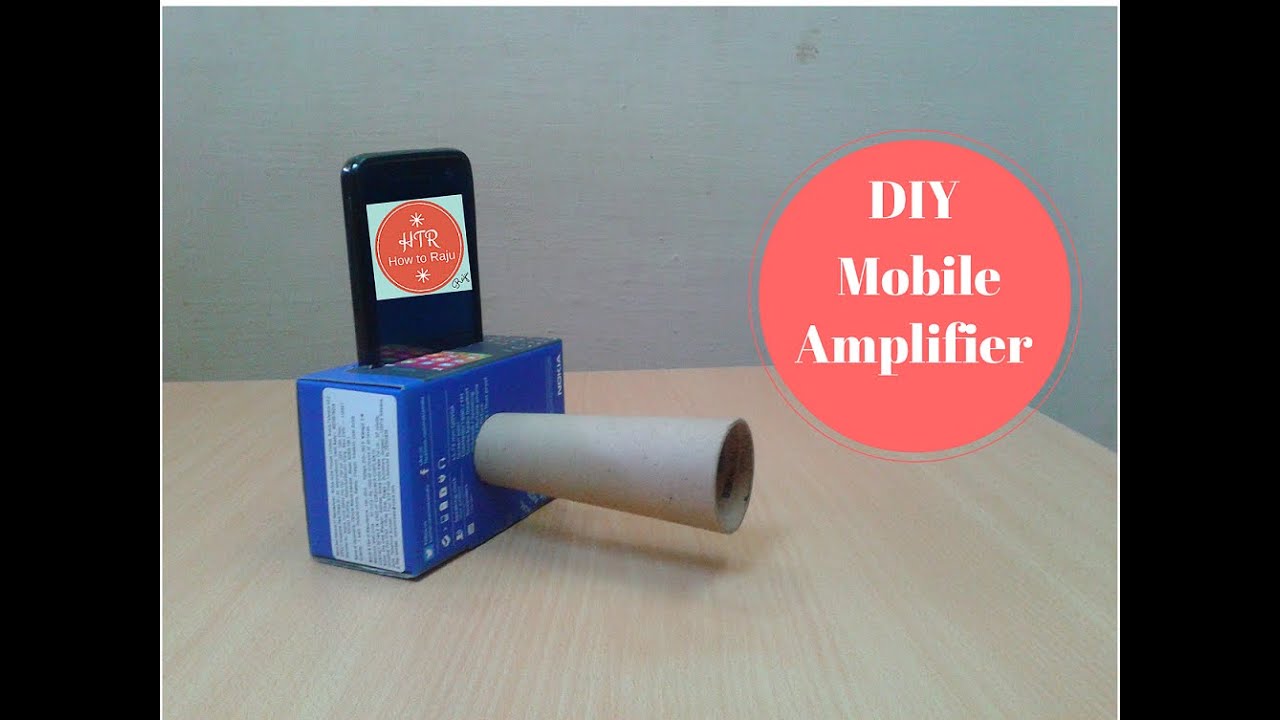
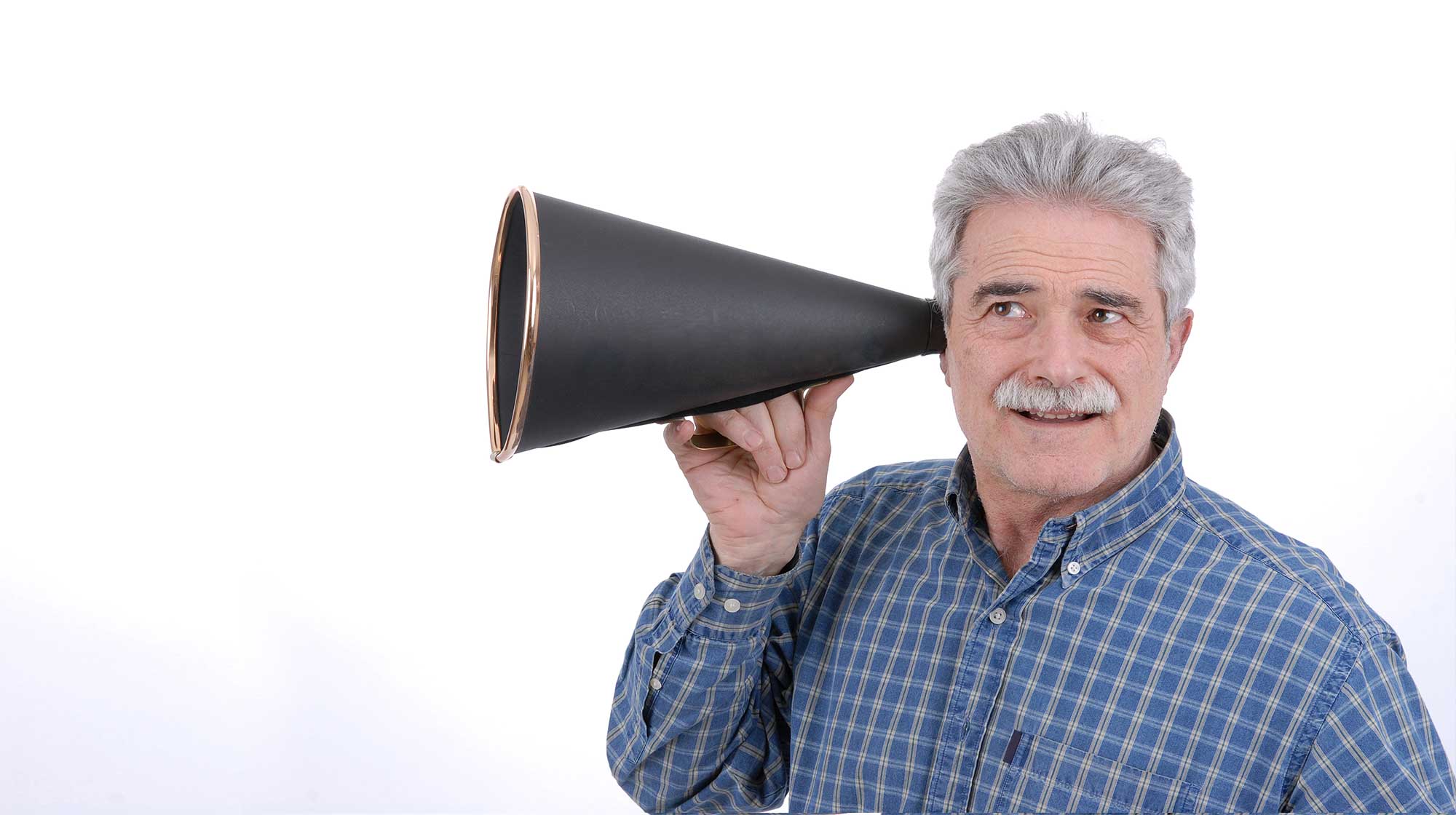
Uniquely among vertebrate sensory receptors, hair cells instead use a mechanical active process to amplify their inputs. Photoreceptors, for example, use a biochemical cascade to enhance their responses several thousandfold after transduction has been accomplished. The receptor cells of most sensory organs must amplify their signals in order to separate them from background noise. In the high-frequency region of the mammalian cochlea, the active process is dominated instead by the phenomenon of electromotility, in which the cell bodies of outer hair cells extend and contract as the protein prestin alters its membrane surface area in response to changes in membrane potential. Taken together, these phenomena explain the four characteristics of the ear’s active process. The active process of non-mammalian tetrapods depends upon active hair-bundle motility, which emerges from the interaction of negative hair-bundle stiffness and myosin-based adaptation motors. These characteristics emerge naturally if the mechanoelectrical transduction process operates near a dynamical instability, the Hopf bifurcation, whose mathematical properties account for specific aspects of our hearing. The inner ear’s performance is greatly enhanced by an active process defined by four features: amplification, frequency selectivity, compressive nonlinearity, and spontaneous otoacoustic emission.
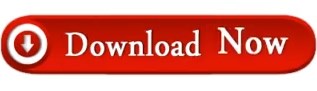